Introduction
Boilers are pressure vessels designed to heat water or produce steam, which can then be used to provide space heating and/or service water heating to a building. In most commercial building heating applications, the heating source in the boiler is a natural gas fired burner. Oil fired burners and electric resistance heaters can be used as well. Steam is preferred over hot water in some applications, including absorption cooling, kitchens, laundries, sterilizers, and steam driven equipment.
Boilers have several strengths that have made them a common feature of buildings. They have a long life, can achieve efficiencies up to 95% or greater, provide an effective method of heating a building, and in the case of steam systems, require little or no pumping energy. However, fuel costs can be considerable, regular maintenance is required, and if maintenance is delayed, repair can be costly.
Guidance for the construction, operation, and maintenance of boilers is provided primarily by the ASME (American Society of Mechanical Engineers), which produces the following resources:
- Rules for construction of heating boilers, Boiler and Pressure Vessel Code, Section IV-2007
- Recommended rules for the care and operation of heating boilers, Boiler and Pressure Vessel Code, Section VII-2007
Boilers are often one of the largest energy users in a building. For every year a boiler system goes unattended, boiler costs can increase approximately 10% (1). Boiler operation and maintenance is therefore a good place to start when looking for ways to reduce energy use and save money.
How Boilers Work
Both gas and oil fired boilers use controlled combustion of the fuel to heat water. The key boiler components involved in this process are the burner, combustion chamber, heat exchanger, and controls.
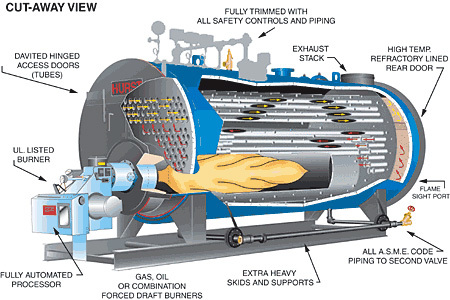
Figure 1: Firetube Boiler (image source: www.hurstboiler.com)
The burner mixes the fuel and oxygen together and, with the assistance of an ignition device, provides a platform for combustion. This combustion takes place in the combustion chamber, and the heat that it generates is transferred to the water through the heat exchanger. Controls regulate the ignition, burner firing rate, fuel supply, air supply, exhaust draft, water temperature, steam pressure, and boiler pressure.
Hot water produced by a boiler is pumped through pipes and delivered to equipment throughout the building, which can include hot water coils in air handling units, service hot water heating equipment, and terminal units. Steam boilers produce steam that flows through pipes from areas of high pressure to areas of low pressure, unaided by an external energy source such as a pump. Steam utilized for heating can be directly utilized by steam using equipment or can provide heat through a heat exchanger that supplies hot water to the equipment.
The discussion of different types of boilers, below, provides more detail on the designs of specific boiler systems.
Types of Boilers
Boilers are classified into different types based on their working pressure and temperature, fuel type, draft method, size and capacity, and whether they condense the water vapor in the combustion gases. Boilers are also sometimes described by their key components, such as heat exchanger materials or tube design. These other characteristics are discussed in the following section on Key Components of Boilers.
Two primary types of boilers include Firetube and Watertube boilers. In a Firetube boiler, hot gases of combustion flow through a series of tubes surrounded by water. Alternatively, in a Watertube boiler, water flows in the inside of the tubes and the hot gases from combustion flow around the outside of the tubes. A drawing of a watertube boiler is shown in Figure 2.
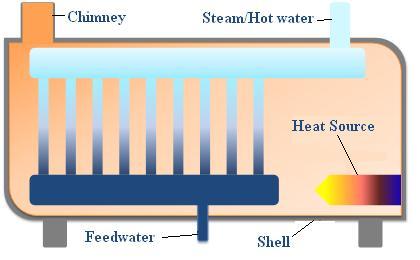
Figure 2: Watertube Boiler
Firetube boilers are more commonly available for low pressure steam or hot water applications, and are available in sizes ranging from 500,000 to 75,000,000 BTU input (5). Watertube boilers are primarily used in higher pressure steam applications and are used extensively for comfort heating applications. They typically range in size from 500,000 to more than 20,000,000 BTU input (5).
Cast iron sectional boilers (figure 3) are another type of boiler commonly used in commercial space heating applications. These types of boilers don’t use tubes. Instead, they’re built up from cast iron sections that have water and combustion gas passages. The iron castings are bolted together, similar to an old steam radiator. The sections are sealed together by gaskets. They’re available for producing steam or hot water, and are available in sizes ranging from 35,000 to 14,000,000 BTU input (2).
Cast iron sectional boilers are advantageous because they can be assembled on site, allowing them to be transported through doors and smaller openings. Their main disadvantage is that because the sections are sealed together with gaskets, they are prone to leakage as the gaskets age and are attacked by boiler treatment chemicals.
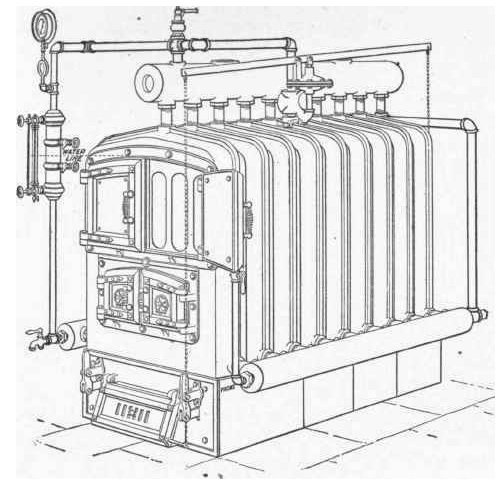
Figure 3: Cast Iron Sectional Boiler (image source: www.chestofbooks.com)
Working Pressure and Temperature
Boilers are classified as either low pressure or high pressure and are constructed to meet ASME Boiler and Pressure Vessel Code requirements. Low-pressure boilers are limited to a maximum working pressure of 15 psig (pound-force per square inch gauge) for steam and 160 psig for hot water (2). Most boilers used in HVAC applications are low-pressure boilers. High-pressure boilers are constructed to operate above the limits set for low-pressure boilers, and are typically used for power generation. Operating water temperatures for hot water boilers are limited to 250o F (2).
Fuel Type
In commercial buildings, natural gas is the most common boiler fuel, because it is usually readily available, burns cleanly, and is typically less expensive than oil or electricity. Some boilers are designed to burn more than one fuel (typically natural gas and fuel oil). Dual fuel boilers provide the operator with fuel redundancy in the event of a fuel supply interruption. They also allow the customer to utilize the fuel oil during “peak time” rates for natural gas. In times when the rates for natural gas are greater than the alternate fuel, this can reduce fuel costs by using the cheaper alternate fuel and limiting natural gas use to occur only during “off peak” times.
Electric boilers are used in facilities with requirements for a small amount of steam or where natural gas is not available. Electric boilers are known for being clean, quiet, and easy to install, and compact. The lack of combustion results in reduced complexity in design and operation and less maintenance. Heating elements are easily replaced if they fail. These types of boilers can be used to produce low or high pressure steam or water, and may be good alternatives for customers who are restricted by emissions regulations. Sizes range from 30,000 to 11,000,000 BTU input with overall efficiency generally in the range of 92% to 96% (2).
Draft Methods
The pressure difference between the boiler combustion chamber and the flue (also called the exhaust stack) produces a draft which carries the combustion products through the boiler and up the flue. Natural draft boilers rely on the natural buoyancy of hot gasses to exhaust combustion products up the boiler flue and draw fresh air into the combustion chamber. Mechanical draft boilers include: Forced Draft, where air is forced into the combustion chamber by a fan or blower to maintain a positive pressure; and Induced Draft, where air is drawn through the combustion chamber by a fan or blower to maintain a negative pressure.
Size and Capacity
Modular Boilers are small in size and capacity and are often intended to replace a large single boiler with several small boilers. These modular boilers can easily fit through a standard doorway, and be transported in elevators and stairways. The units can be arranged in a variety of configurations to utilize limited space or to accommodate new equipment. Modular boilers can be staged to efficiently meet the demand of the heating load.
Condensing Method
Traditional hot water boilers operate without condensing out water vapor from the flue gas. This is critical to prevent corrosion of the boiler components. Condensing Boilers operate at a lower return water temperature than traditional boilers, which causes water vapor to condense out of the exhaust gasses. This allows the condensing boiler to extract additional heat from the phase change from water vapor to liquid and increases boiler efficiency. Some carbon dioxide dissolves in the condensate and forms carbonic acid. While some condensing boilers are made to handle the corrosive condensation, others require some means of neutralizing the condensate. Traditional non-condensing boilers typically operate in the range of 75% – 86% combustion efficiency, while condensing boilers generally operate in the range of 88% to 95% combustion efficiency (2).
Key Components of Boilers
The key elements of a boiler include the burner, combustion chamber, heat exchanger, exhaust stack, and controls. Boiler accessories including the flue gas economizer are also commonly used as an effective method to recover heat from a boiler and will be discussed briefly in the section Best Practices for Efficient Operation.
Natural gas boilers employ one of two types of burners, atmospheric burners, also called natural draft burners and forced draft burners, also called power burners. Due to more stringent federal and state air quality regulations, low NOx burners and pre-mix burners are becoming more commonly used and even required in some areas. By ensuring efficient mixing of air and fuel as it enters the burner, these types of burners can ensure that NOx emissions are reduced.
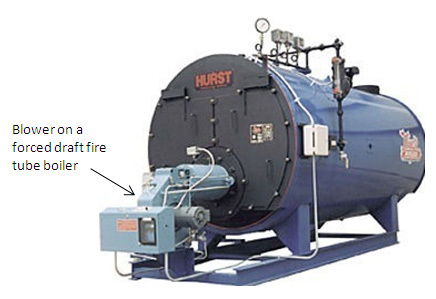
Figure 4: Forced Draft Boiler (image source: www.Hurstboiler.com)
The combustion chamber, usually made of cast iron or steel, houses the burners and combustion process. Temperatures inside the combustion chamber can reach several hundred degrees very quickly.
Heat exchangers may be made from cast iron, steel tube bundles, or, in the case of some smaller boilers, copper or copper-clad steel.
The exhaust stack or flue is the piping that conveys the hot combustion gasses away from the boiler to the outside. Typically this piping is made of steel, but in the case of condensing boilers it needs to be constructed of stainless steel to handle the corrosive condensate. Another consideration is whether the exhaust stack will be under a positive or negative pressure. This can determine how the joints of the exhaust stack must be sealed.
Boiler controls help produce hot water or steam in a regulated, efficient, and safe manner. Combustion and operating controls regulate the rate of fuel use to meet the demand. The main operating control monitors hot water temperature or steam pressure and sends a signal to control the firing rate, the rate at which fuel and air enters the burner. Common burner firing sequences include on/off, high/low/off and modulating.
Boiler safety controls include high pressure and temperature, high and low gas/oil pressure, and high and low water level and flame safeguard controls. These controls are considered safeties or limits that break the electrical circuit to prevent firing of the boiler. For example, in the event pressure in the boiler exceeds the pressure limit setting, the fuel valve is closed to prevent an unsafe, high pressure condition. The safety circuit of a flame safeguard control system typically includes switch contacts for low water cutoff, high limits, air proving switches, redundant safety and operating controls, and flame detectors. Flame detectors often consist of flame rods, and ultraviolet or infrared scanners to monitor the flame condition and deactivate the burner in the event of a non ignition or other unsafe condition. Flame safeguard controls are programmed to operate the burner and cycle it through the stages of operation.
Safety Issues
All combustion equipment must be operated properly to prevent dangerous conditions or disasters from occurring, causing personal injury and property loss. The basic cause of boiler explosions is ignition of a combustible gas that has accumulated within the boiler. This situation could arise in a number of ways, for example fuel, air, or ignition is interrupted for some reason, the flame extinguishes, and combustible gas accumulates and is reignited. Another example is when a number of unsuccessful attempts at ignition occur without the appropriate purging of accumulated combustible gas.
There is a tremendous amount of stored energy within a boiler. The state change of superheated water from a hot liquid to a vapor (steam) releases an enormous amount of energy. For example, 1 ft3 of water will expand to 1600 ft3 when it turns to steam. Therefore, “if you could capture all the energy released when a 30 gallon home hot water tank flashes into explosive failure at 332oF, you would have enough force to send the average car (weighing 2,500 lbs) to a height of nearly 125 feet. This is equivalent to more than the height of a 14 story apartment building, starting with a lift off velocity of 85 miles per hour!” (5).
Boiler safety is a key objective of the National Board of Boiler and Pressure Vessel Inspectors. This organization reports and tracks boiler safety and the number of incidents related to boilers and pressure vessels each year. Their work has found that the number one incident category resulting in injury was poor maintenance and operator error (5). This stresses the importance of proper maintenance and operator training.
Boilers must be inspected regularly based on manufacturer’s recommendations. Pressure vessel integrity, checking of safety relief valves, water cutoff devices and proper float operation, gauges and water level indicators should all be inspected. The boiler’s fuel and burner system requires proper inspection and maintenance to ensure efficient operation, heat transfer and correct flame detection. The Federal Energy Management Project (FEMP) O&M Best Practices Guide to Achieving Operation Efficiency is a good resource describing a preventive maintenance plan and also explaining the importance of such a plan. This document is available here: http://www1.eere.energy.gov/femp/pdfs/omguide_complete.pdf.
Best Practices for Efficient Operation
Efficiency
The percentage of the heat energy contained in the fuel that is captured by the working fluid (e.g. water) in the boiler is defined as the combustion efficiency of the boiler. Combustion efficiencies of 80% or higher are usually possible for hot water boilers and low pressure steam boilers for commercial buildings.
Complete combustion results when a hydrocarbon fuel such as natural gas or oil burns and produces only carbon dioxide, water and heat. If there is insufficient oxygen and/or poor mixing of fuel and oxygen, then incomplete combustion will occur resulting in other products of combustion including carbon monoxide and unburned fuel.
When incomplete combustion occurs, the chemical energy of the fuel is not completely released as heat and the combustion efficiency is reduced. This is also a safety concern as unburned fuel could ignite in the stack and cause an explosion. Boilers must be tuned to achieve complete combustion. One strategy to ensure complete combustion is to provide some amount of excess air. However, as shown in the figure below, a small amount of excess air will improve combustion efficiency, but a large amount will reduce efficiency.
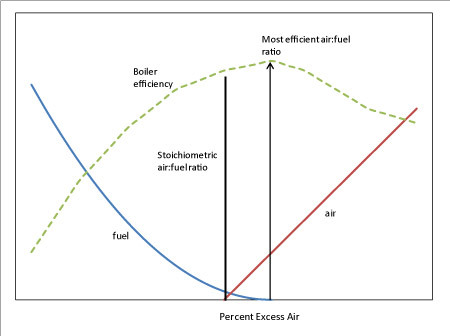
Figure 5: Combustion Efficiency vs. Excess Air
For high overall boiler efficiency, the heat released by combustion must be efficiently transferred into the working fluid. Any heat not transferred into the fluid will be lost through the boiler shell or the flue gas. The temperature of the flue gasses in the boiler stack is a good indicator of this heat transfer and thus the efficiency. There are practical limits to how low the stack temperature can be. The temperature will be higher than the working fluid in the boiler. In non-condensing boilers, it must be high enough so that the water vapor in the exhaust gas does not condense and bathe the heat transfer surface in the corrosive condensate. Condensing natural gas boilers are designed and built with materials designed to resist corrosion. As such, they may have exhaust temperatures less than 150°F. Capturing the heat from the condensate can result in combustion efficiencies of greater than 90%.
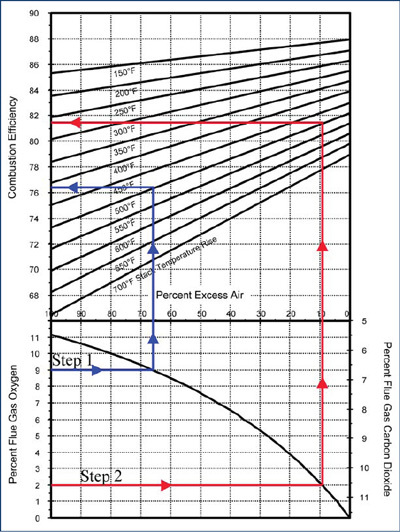
Figure 6: Combustion Efficiency Chart for Natural Gas (image source: Federal Energy Management Program, U.S. Department of Energy)
Figure 6 is a combustion efficiency chart for natural gas fuel with power burners that shows the relationship between excess air, flue gas temperature, and combustion efficiency. As an example, tracing the Step 1 line, at 9% flue gas oxygen (equivalent to about 67% excess air as seen in the graph) and 500oF flue gas temperature rise, the corresponding combustion efficiency is about 76.5%. Using the same 500oF flue gas temperature rise, Step 2 illustrates that dropping to 2% flue gas oxygen results in an improved combustion efficiency of about 81.5%. This is shown as Step 2 in Figure 6 above. As percent flue gas oxygen decreases, less heat is transferred to the excess oxygen, and the combustion efficiency increases. As combustion efficiency increases, more heat is transferred to the feedwater instead of the flue gas, and therefore the flue gas temperature decreases.
Use Boiler Controls for Optimized Air-to-Fuel Ratio
To ensure that complete combustion occurs, extra air is introduced at the burner. But too much will result in air being wastefully heated and exhausted out of the boiler flue, penalizing combustion efficiency, and creating a safety issue. When a boiler is tuned, the goal is to maximize combustion efficiency by providing just enough excess air to assure complete combustion but not too much to reduce efficiency. How much excess air is enough to assure complete combustion? That varies with the design and condition of the burner and boiler, as well as with the different firing rates of the burner, but is typically considered to be between 2% - 3%. Excess air must also be adjusted to allow for variations in temperature, density, and humidity of the boiler combustion air throughout any daily and seasonal variations. It’s desirable to maintain a constant amount of excess air across the entire firing range.
The important idea to remember is that complete combustion is critical to ensuring efficient boiler operation. Incomplete combustion of the fuel can significantly reduce boiler efficiency by 10% or more, while increasing excess air by 10% may only impact boiler efficiency by about 1%. Signs of incomplete combustion are a smoky exhaust, a yellow flame, flame failures, and sooty boiler tubes. It is a good idea to tune up a boiler annually to ensure the combustion process is optimized.
Typically, excess air of around 10% for a natural gas boiler is optimal to ensure complete combustion and peak efficiency. This corresponds to excess O2 of around 2% to 3%. Operating with excess air beyond 10% is undesirable, as it can result in reduced efficiency and higher emissions. Therefore maintaining the optimum level of excess air across the entire firing range is preferred. This can be accomplished with the use of burner controls including parallel positioning controls, cross-limiting controls, and oxygen trim controls. These types of controls are superior alternatives to traditional mechanical jackshaft controls. A brief description of each burner control type is provided below (3):
- Mechanical jackshaft control is the simplest type of modulating burner control, typically used on smaller burners. Also called single point control because one mechanical linkage assembly controls both air and fuel. These controls cannot measure airflow or fuel flow. The range of control is limited, resulting in excessive levels of excess air to ensure safe operation under all conditions and firing rates. Slop in the linkages makes accurate and repeated control difficult, and requires regular maintenance and adjustment.
- Parallel positioning controls use separate motors to adjust fuel flow and airflow allowing each to be adjusted over the entire firing range of the boiler. During setup, many points are “mapped,” typically 10 to 25 points, to create a curve of airflow and corresponding fuel flow. The air-fuel ratio can therefore vary across the entire firing range to provide the optimal ratio under all firing conditions. Also, with the use of electronic servo-motors, this method of control is highly repeatable.
- Cross-limiting controls, usually applied to larger boilers, use controls to sense and compensate for some of the factors that affect optimum air to fuel ratio. Air flow and fuel flow are measured and adjusted to maintain the optimum value determined during initial calibration.
- Oxygen trim control is used in conjunction with standard parallel positioning or cross-limiting controls. It analyzes the oxygen in the flue gas and adjusts the air-fuel ratio accordingly to maintain a set amount of excess oxygen. These controls are usually installed on larger boilers with high annual fuel usage, and can increase energy efficiency by one or two percent beyond what is achieved with the standard control alone.
Monitor Boiler Gauges
It is possible that a leak will develop in the hot water distribution loop. Such leaks will increase the system’s energy and water consumption, and may also result in water damage. Hot water and steam distribution systems should be provided with make-up water to replace any steam or water that is lost through a leak in the system. This will provide an easy way to ensure the system is fully charged with water at all times. It is best practice to install a meter on the make-up line to the system. The meter should be read weekly to check for unexpected losses of water from the system.
In steam systems, it is a best practice to monitor make-up water volume daily. As steam leaks from the system, additional make-up water is required to replace the loss. Monitoring the make-up water will ensure that you are maximizing the return of condensate, thereby reducing the need for make-up water.
Seasonal Operation
If a steam or hot water system is not used for a portion of the year, shutting the system down can result in significant savings. Maintaining a boiler at its operating temperature consumes energy equivalent to its standby losses. In the case of a hot water system, energy use may also include pump operation.
Operating Multiple Boiler Plants
Boiler loads in commercial buildings vary greatly from summer to winter, from day to night, and from weekday to weekend. With a single boiler it is difficult to efficiently supply these varying loads. When the building heating needs drop below the heat supplied by the boiler at its lowest firing rate, the boiler cycles off. Cycling a boiler on and off is very inefficient because there is a pre-ignition purge and a post-ignition purge that draw heat out of the boiler with each cycle. Also, in the case of a non-modulating boiler, cycling doesn’t allow the boiler to operate at part load and steady firing rate when combustion efficiencies are at their best.
If a facility has multiple boilers, it may be possible to sequence the boilers to avoid frequent cycling. If using non-modulating boilers, it may be better to stage subsequent boilers on once the primary boiler has reached full capacity, rather than cycling multiple boilers on and off to meet the load. On the other hand, with modulating boilers, boiler efficiency increases at part load conditions. Therefore it may be advantageous to operate multiple boilers simultaneously at part load conditions rather than one boiler at 100% output. Figure 7 below shows the relationship between firing rate and efficiency for a boiler with the ability to modulate both airflow and fuel input.
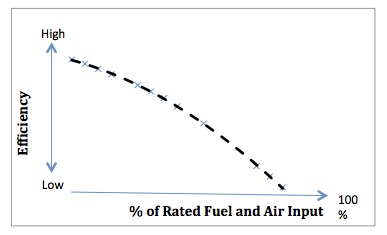
Figure 7: Efficiency as a Function of Fuel and Air Input for Modulating Boilers
Finally, automatic sequencing of boilers is essential to efficient operation. When building loads are reduced at night and on weekends, increased boiler cycling will likely occur if no-one is available to turn boilers off as necessary.
If your facility has multiple boilers you should assess if it is truly necessary to keep any boilers on standby (at pressure or temperature), since this has an energy penalty. The standby boiler will not only cycle on and off, it will lose heat to the surroundings through radiation losses, which increase significantly as a percentage of boiler input at reduced firing rates. At low firing rates, such as when a boiler is maintained in a standby condition, efficiency loss can be as much as 15% (7). Having a standby boiler will allow quick recovery if the lead boiler fails, but this must be weighed against this large energy penalty. If a standby boiler is not critical to your operation, or if the need for a standby boiler is seasonal, you should consider shutting off any unnecessary boilers to prevent these energy losses.
Implement Boiler Lockout Control Sequence
Including boiler lockout in the HVAC system’s sequence of operation is important in achieving energy efficiency. With the common application of VAV systems in commercial buildings today, simultaneous heating and cooling, and excessive reheating of primary air can often go unnoticed. Implementing a boiler lockout based on outside air temperature, for example when outside air is greater than 65oF, is an effective way to prevent these conditions.
Condensing Boilers
Both the system design and operating conditions are critical to the successful operation and performance of a condensing boiler. Return water temperatures below 130oF are typically required to get the rated efficiency out of a condensing boiler. Return water temperatures above 130oF prevent condensation of the flue gas and result in the boiler operating no more efficiently than a traditional boiler.
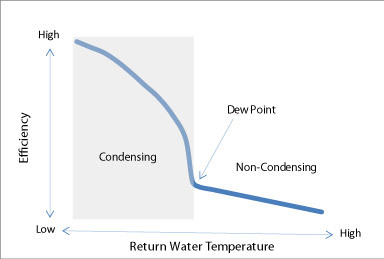
Figure 8: Effect of Return Water Temperature on Efficiency of Condensing Boilers
Flue Gas Economizers
Flue gas economizers offer the best opportunity for heat recovery (3). These are essentially heat exchangers in the boiler exhaust which transfer heat from the flue gas to either the boiler feedwater or combustion air. Even with efficient boilers that operate with a relatively low flue gas temperature, there is ample room to recover some of the flue gas heat that would otherwise go up the stack. Economizers typically increase overall boiler efficiency by three to four percent (3).
Best Practices for Maintenance
Keep the Boiler Clean
As mentioned previously, any residue, such as soot or scale, that coats the heat transfer surfaces of the boiler will reduce its efficiency and also increase the likelihood of equipment failure. Cleaning this surface according to manufacturer’s recommendations is important to maintaining optimum boiler performance and equipment life. Residue that coats the tubes of a boiler will interfere with heat transfer and elevate the flue gas temperature. If incomplete combustion occurs, the resulting soot accumulates on the combustion side of the tubes. Similarly, poor water treatment practices can result in scale accumulation on the water side of the tubes. A layer of soot or scale only 0.03 inches thick can reduce heat transfer by 9.5%. A layer 0.18 inches thick can reduce heat transfer by 69%. (3).
Water Chemical Treatment Plan
Good boiler water chemical treatment is essential to maintain efficient operation. Each chemical treatment plan must be customized based on the dissolved minerals in the make-up water, the percentage of condensate returned, and the presence or absence of a de-aerator. Dissolved solids in the boiler water and the level of treatment chemicals should be tested daily in small low pressure plants and hourly in larger high pressure plants. Instruments should be calibrated monthly. Annual inspections of boilers should include a thorough examination of the water side surfaces for evidence of scaling and corrosion. Even a thin layer of scale interferes with heat transfer and thereby decreases combustion efficiency.
An upward trend in flue gas temperatures over weeks or months usually indicates that a deposit has built up on either the fireside or waterside of the boiler heat exchange surfaces. If this condition is observed the boiler should be inspected promptly.
Minimize Boiler Blowdown
Having too many total dissolved solids (TDS’s) in the boiler water can cause scale and reduce boiler efficiency. Therefore, it is necessary to maintain the solids below certain limits. As TDS concentration increases, it becomes more likely that the dissolved solids will precipate out of the water and form scale. Draining of the water, called boiler blowdown, is required to remove some of those dissolved solids and keep the TDS concentration below the level where they will precipitate. Consistent and frequent small volume blowdowns is a better practice than infrequent high volume blowdowns, because it conserves energy, water, and chemicals. Large steam boilers with steady loads should have continuous blowdown, where a small amount of water is drained continuously from the boiler while fresh make-up water is introduced.
Inspect and Repair Insulation
Insulation is critical for steam and condensate piping. Un-insulated pipes, valves, or fittings carry a heavy energy penalty. It’s generally cost effective to insulate any surface with a temperature greater than 130oF (4). Steam, condensate, and hot water pipes in air conditioned spaces produce a double penalty if un-insulated because the heat loss from the pipes must be removed by additional air conditioning.
Sample Maintenance Logs & Boiler Checklists
Boiler O&M best practices begin with maintaining regularly scheduled inspection logs and checklists to ensure proper equipment operation. Pressure, water temperature, and flue gas temperatures should be recorded daily, as they can serve as a baseline reference for system operation and troubleshooting problems. More detailed inspections and checks should be performed to document system performance, which can be very important since a gradual change in system operating conditions over time may not be readily apparent without the use of such documentation. The Federal Energy Management Program O&M Best Practices Guide to Achieving Operational Efficiency (5) contains example Daily, Weekly, and Monthly Maintenance and Inspection Logs that can be adapted to your facility. The following Maintenance Checklists have been assembled based on the recommended best practices found in this document as well.
Table 1: Sample Daily Boiler Checklist
Description | Comments | Maintenance Frequency | |||
Daily | Weekly | Monthly | Annually | ||
Boiler use/sequencing | Turn off/sequence unnecessary boilers | X | |||
Overall visual inspection | Complete overall visual inspection to be sure all equipment is operating and safety systems are in place | X | |||
Follow manufacturer’s recommended procedures in lubricating all components | Compare temperatures with tests performed after annual cleaning | X | |||
Check steam pressure | Is variation in steam pressure as expected under different loads? Wet steam may be produced if the pressure drops too fast | X | |||
Check unstable water level | Unstable levels can be a sign of contaminates in feedwater, overloading of boiler, equipment malfunction | X | |||
Check burner | Check for proper control and cleanliness | X | |||
Check motor condition | Check for proper function temperatures | X | |||
Check air temperatures in boiler room | Temperatures should not exceed or drop below design limits | X | |||
Boiler blowdown | Verify the bottom, surface and water column blow downs are occurring and are effective | X | |||
Boiler logs | Keep daily logs on: • Type and amount of fuel used • Flue gas temperature • Makeup water volume • Steam pressure, temperature, and amount generated Look for variations as a method of fault detection | X | |||
Check oil filter assemblies | Check and clean/replace oil filters and strainers | X | |||
Inspect oil heaters | Check to ensure that oil is at proper temperature prior to burning | X | |||
Check boiler water treatment | Confirm water treatment system is functioning properly | X |
Table source: Federal Energy Management Program, O&M Best Practices Guide to Achieving Operational Efficiency
Table 2: Sample Weekly Boiler Checklist
Description | Comments | Maintenance Frequency | |||
Daily | Weekly | Monthly | Annually | ||
Check flue gas temperatures and composition | Measure flue gas composition and temperatures at selected firing positions – recommended O2% and CO2% Fuel O2% CO2% Natural gas 1.5 10 No. 2 fuel oil 2.0 11.5 No. 6 fuel oil 2.5 12.5 Note: percentages may vary due to fuel composition variations | X | |||
Check all relief valves | Check for leaks | X | |||
Check water level control | Stop feedwater pump and allow control to stop fuel flow to burner. Do not allow water level to drop below recommended level. | X | |||
Check pilot and burner assemblies | Clean pilot and burner following manufacturer’s guidelines. Examine for mineral or corrosion buildup. | X | |||
Check boiler operating characteristics | Stop fuel flow and observe flame failure. Start boiler and observe characteristics of flame. | X | |||
Inspect system for water/ steam leaks and leakage opportunities | Look for: leaks, defective valves and traps, corroded piping, condition of insulation | X | |||
Inspect all linkages on combustion air dampers and fuel valves | Check for proper setting and tightness | X | |||
Inspect boiler for air leaks | Check damper seals | X |
Table source: Federal Energy Management Program, O&M Best Practices Guide to Achieving Operational Efficiency
Table 3: Sample Monthly Boiler Checklist
Description | Comments | Maintenance Frequency | |||
Daily | Weekly | Monthly | Annually | ||
Check blowdown and water treatment procedures | Determine if blowdown is adequate to prevent solids buildup | X | |||
Flue gases | Measure and compare last month’s readings flue gas composition over entire firing range | X | |||
Combustion air supply | Check combustion air inlet to boiler room and boiler to make sure openings are adequate and clean | X | |||
Check fuel system | Check pressure gauge, pumps, filters and transfer lines. Clean filters as required. | X | |||
Check belts and packing glands | Check belts for proper tension. Check packing glands for compression leakage. | X | |||
Check for air leaks | Check for air leaks around access openings and flame scanner assembly. | X | |||
Check all blower belts | Check for tightness and minimum slippage. | X | |||
Check all gaskets | Check gaskets for tight sealing, replace if do not provide tight seal | X | |||
Inspect boiler insulation | Inspect all boiler insulation and casings for hot spots | X | |||
Steam control valves | Calibrate steam control valves as specified by manufacturer | X | |||
Pressure reducing/regulating | Check for proper operation valves | X | |||
Perform water quality test | Check water quality for proper chemical balance | X |
Table source: Federal Energy Management Program, O&M Best Practices Guide to Achieving Operational Efficiency
Table 4: Sample Annual Boiler Checklist
Description | Comments | Maintenance Frequency | |||
Daily | Weekly | Monthly | Annually | ||
Clean water side surfaces | Follow manufacturer’s recommendation on cleaning and preparing water side surfaces | X | |||
Clean fire side | Follow manufacturer’s recommendation on cleaning and preparing fire side surfaces | X | |||
Inspect and repair refractories on fire side | Use recommended material and procedures | X | |||
Check fuel system | Check pressure gauge, pumps, filters and transfer lines. Clean filters as required. | X | |||
Relief valve | Remove and recondition or replace | X | |||
Feedwater system | Clean and recondition feedwater pumps. Clean condensate receivers and deaeration system | X | |||
Fuel system | Clean and recondition system pumps, filters, pilot, oil preheaters, oil storage tanks, etc. | X | |||
Electrical systems | Clean all electrical terminals. Check electronic controls and replace any defective parts. | X | |||
Hydraulic and pneumatic valves | Check operation and repair as necessary | X | |||
Flue gases | Make adjustments to give optimal flue gas composition. Record composition, firing position, and temperature. | X | |||
Eddy current test | As required, conduct eddy current test to assess tube wall thickness | X |
Table source: Federal Energy Management Program, O&M Best Practices Guide to Achieving Operational Efficiency
References
- Capehart, B., Turner, W. and Kennedy, W., 2006. Guide to Energy Management.
- ASHRAE Handbook, HVAC Systems and Equipment, 2008.
- Boiler and Heaters, Improving Energy Efficiency, Canadian Industry Program for Energy Conservation, August 2001. http://oee.nrcan.gc.ca/publica...
- Federal Energy Management Program Fact Sheet, PNNL, January 2005. http://www1.eere.energy.gov/femp/pdfs/om_combustion.pdf
- FEMP O&M Best Practices, a Guide to Achieving Operational Efficiency, U.S. Department of Energy, August 2010. http://www1.eere.energy.gov/femp/pdfs/omguide_complete.pdf
- Efficient Boiler Operations Sourcebook, Fourth Edition, F. William Payne and Richard E. Thompson, 1996.
- The Control of Boilers, 2nd Edition, Sam G. Dukelow, 1991.
Other Resources
- The National Board of Boilers and Pressure Vessel Inspectors, http://www.nationalboard.org/default.aspx.
- 2010 ASME Boiler and Pressure Vessel Code (BPVC), http://go.asme.org/bpvc10.